Flood-tolerant rice for enhanced production and livelihood of smallholder farmers of Africa
- 1Department of Crop Science and Horticulture, College of Agriculture, Sokoine University of Agriculture, Morogoro, Tanzania
- 2Department of Research and Innovation, Tanzania Agricultural Research Institute, Kigoma, Tanzania
- 3Rice Breeding Innovations Department, International Rice Research Institute, Los Baños, Philippines
- 4Department of Biology, University of Copenhagen, Copenhagen, Denmark
Climate change has intensified food security challenges, especially in Africa, where a significant portion of produce is reliant on smallholder farmers in rainfed conditions. Prolonged flooding and droughts, driven by erratic weather patterns, have significantly elevated the risk of food scarcity. Floods, in particular, have been responsible for severe crop losses, raising concerns about increasing import costs if this issue is not mitigated. Africa is actively working to mitigate the impacts of flooding and enhance food security, although progress has been gradual. Developing flood-resilient varieties is a promising strategy to address this challenge. We explored various flood types common in the region and observed a scarcity of research on flood-resilient varieties, particularly those adapted for anaerobic germination and stagnant flooding. Conversely, varieties bred for flash flooding, such as FARO 66 and FARO 67, have seen limited distribution, primarily confined to a few West African countries, falling short of the intended impact. In contrast, deepwater tolerance research dates back to the early 1900s, but commercialization of the varieties remains limited, with scarce information regarding their cultivation, coverage, and performance. Newly developed varieties, such as Kolondieba 2 and Kadia 24, have received less attention, leaving many farmers dependent on locally adapted cultivars specific to particular areas. Remarkably, despite the limited information, both released and local stress-tolerant cultivars exhibit substantial survival rates and yield advantages. For instance, FARO 66 and FARO 67 have demonstrated 1–3 t/ha yield advantages over recurrent parents under flooding stress. Nonetheless, further efforts are required to address various forms of flooding. To this end, AfricaRice collaborates with National Rice Development Strategies, IRRI, and other partners to promote research and development. While improved flood-tolerant varieties remain limited in scope across Africa, the financial gains for farmers are significant when compared to susceptible cultivars. As the continent’s population continues to grow rapidly, there is untapped potential in African germplasms, making ongoing research and breeding strategies essential. Therefore, this review highlights the importance of intensifying efforts in screening and identifying flood-tolerant rice. Furthermore, it underscores the value of utilizing traditional flood-resilient cultivars in breeding to enhance the productivity of widely distributed and cultivated varieties.
1 Introduction
Rice serves as a dietary cornerstone for over half of the world’s population (Muthayya et al., 2014). It contributes significantly, accounting for approximately 23% of the daily caloric intake (Chemutai et al., 2016). Asia has historically dominated rice production, with China and India jointly responsible for over 90% of the world’s rice output (Fukagawa and Ziska, 2019). Nevertheless, substantial changes have been observed in Africa, driven by shifts in dietary preferences, population growth, and urbanization. Tsujimoto et al. (2019) highlight a significant surge in rice consumption, from 9.2 Mt. in 1990 to 31.5 Mt. in 2019. This rapid growth in consumption is beginning to strain production capacities. Ibrahim et al. (2022) reported that between 2009 and 2019, Sub-Saharan Africa’s (SSA) average rice consumption stood at 27.4 Mt., surpassing the average production of 15.4 Mt. over the same period.
This persistent deficit between production and consumption has made Africa heavily reliant on rice imports, incurring substantial costs [Africa Rice Center (AfricaRice), 2011]. Over the past five decades, Africa has experienced a notable increase in rice production, primarily attributed to the expansion of cultivated areas rather than substantial improvements in productivity (FAOSTAT, 2012). Africa’s average rice yield lags significantly behind that of Asia, with African farmers harvesting an average of 2.28 tonnes per hectare (t/ha) compared to Asia’s 4.61 t/ha (Arouna et al., 2021). This productivity gap is particularly pronounced among small-scale resource-poor farmers who practice rainfed agriculture (Hong et al., 2021). Africa Rice Center (AfricaRice) (2011) asserts that addressing these constraints is essential to curb rice import dependence, which can be achieved through research and development efforts.
Some of the major challenges for rice farmers across Africa are flooding and prolonged dry spells. These challenges have become more frequently pronounced as the largest African production areas are located in rainfed environments that suffer from weather variabilities [Diagne et al., 2013b; Africa Rice Center (AfricaRice), 2019]. These abiotic constraints interact with harsh weather conditions, amplifying their detrimental effects. Recent data from Hong et al. (2021) emphasize that small-scale farmers are responsible for more than 90% of rice output in Africa. Rainfed farming dominates rice production in most Sub-Saharan African countries (Dramé et al., 2013), with nearly 70% of rice production occurring under rainfed conditions (Ibrahim et al., 2022). Rainfed lowlands, in particular, contribute significantly to rice production in Africa, encompassing approximately 37% of cultivated areas and yielding 48% of the production [Africa Rice Center (AfricaRice), 2011].
However, the full potential of rainfed lowlands remains untapped due to challenges related to the adverse effects of floods (Agbeleye et al., 2019). It is evident that African major rice-growing areas are flood prone (Kuya et al., 2019), with varying water levels, crop stages during floodwater occurrence, and durations of water accumulation. Moreover, with the existence of various ecosystem types, even the types of flooding are different; some areas experience more than one type. These types commonly include floods at germination, flash floods, partial submergence, and prolonged deep water (Agbeleye et al., 2019). It is devastating that the impacts of flooding are capable of causing total crop losses, thus leading to famine. Therefore, addressing the challenges posed by floods is imperative to enhance rice productivity and expand rice cultivation areas, particularly in rainfed lowlands.
Developing high-yielding, flood-tolerant rice is essential for ensuring resilience in rice farming (Bairagi et al., 2021). While extensive research has been conducted globally, there is limited and scattered research specific to Africa. Mackill et al. (2012) have reported the promising adoption of flood-tolerant varieties by the majority of farmers in Asia. However, this is not the case for Africa, where there are few reports regarding the adoption, distribution, and use of varieties capable of withstanding inundation. The intriguing scenario is that the continent possesses a wealth of rice cultivars suitable for genetic improvement. This offers promise for breeding efforts in the face of escalating weather challenges. Therefore, to potentially harness the rice-growing areas where floods are common, it is imperative to publicly reveal the current measures and future directions for developing flood-tolerant rice. As a result, this review aims to present Africa’s current progress in addressing the various types of flooding stresses in rice cultivation.
2 Overview of rice sector in Africa
2.1 Rice area and productivity
Rice plays a vital role in ensuring food availability and income security for both rural and urban populations in Africa. Smallholder farmers are at the forefront of rice production, with more than 80% of the total production in Africa attributed to them (Sie et al., 2012; Tanaka et al., 2017). The expansion of rice cultivation has shown varying trends over the years, and it is evident that the increase in production is primarily linked to the expansion of cultivation areas. Balasubramanian et al. (2007) pointed out that there is an immense potential for rice cultivation in Africa, with approximately 239 million hectares of potential wetlands available. However, it is unfortunate that less than 5% of this land is currently utilized for rice cultivation. A report by the FAO in 2008, cited by Rodenburg and Johnson (2009), indicated a significant 105% increase in harvested rice area over three decades, which was driven by the growing demand for rice.
According to Africa Rice Center (AfricaRice) (2011), the total area utilized for rice production in Africa was around 10 million hectares, encompassing all African countries engaged in rice cultivation. Nigatu et al. (2017) highlighted that in 2016, the harvested rice area in Sub-Saharan Africa reached 11.2 million hectares, representing a 4.2% increase between 2010 and 2016. However, the notable increase in production was primarily attributed to the expansion of cultivation areas rather than improvements in yield per hectare. In 2010, rice productivity in Africa was reported to be 2.1 t/ha, and this yield remained relatively consistent between 2014 and 2016 (Nigatu et al., 2017). Other studies by Roy-Macauley (2018) and Tsujimoto et al. (2019) reported similar rice yields in the range of 2.1 to 2.35 t/ha for Sub-Saharan Africa. A more recent study by Arouna et al. (2021) estimated the average rice yield in Africa to be 2.28 t/ha, while Rodenburg and Saito (2022) indicated that Africa’s rice yield is around 2 t/ha.
Comparing these yield figures to the potential yield gap of 2–10 t/ha reveals that Africa is currently achieving only a fraction of its rice production potential. Addressing the constraints that limit production is crucial for improving the supply of rice in Africa and closing this yield gap.
2.2 Major producers
The trends in rice production in Africa reveal a general increase in production, although this increase has not kept pace with the growing demand for rice. Longtau (2003) highlighted that West Africa, in particular, had the highest production and consumption of rice in Africa, accounting for 64.2% of production and 61.9% of consumption. Notable rice-producing countries in Sub-Saharan Africa (SSA) identified by Rodenburg and Johnson (2009) include Nigeria, Madagascar, Guinea, Sierra Leone, Egypt, the Democratic Republic of Congo, Mali, Côte d’Ivoire, Tanzania, and Mozambique. Mutiga et al. (2021) and Nigatu et al. (2017) noted that nearly 80% of rice production and consumption in SSA can be attributed to Nigeria, Madagascar, Côte d’Ivoire, Tanzania, Mali, Guinea, Sierra Leone, and Senegal.
Recent data from FAOSTAT (2023), which provides information on harvested rice area and production, confirm that West Africa has the largest harvested rice area and highest actual production among the five African regions (Table 1). In contrast, Southern Africa recorded the lowest rice area and production. Nigeria stands out as the leading rice producer in Africa, surpassing all other countries by a significant margin. In 2020, Nigeria’s rice production reached 8.17 Mt., making it the largest producer on the continent. Egypt, Madagascar, Tanzania, and Mali also feature among the top rice-producing countries (Table 2). While some countries experienced a decline in rice production between 2010 and 2015, such as Madagascar, Tanzania, and Sierra Leone, most of Africa’s major rice-producing nations saw an increase in production during this period. From 2015 to 2020, only Côte d’Ivoire and Egypt reported a reduction in rice production among the ten selected major rice producers in Africa.
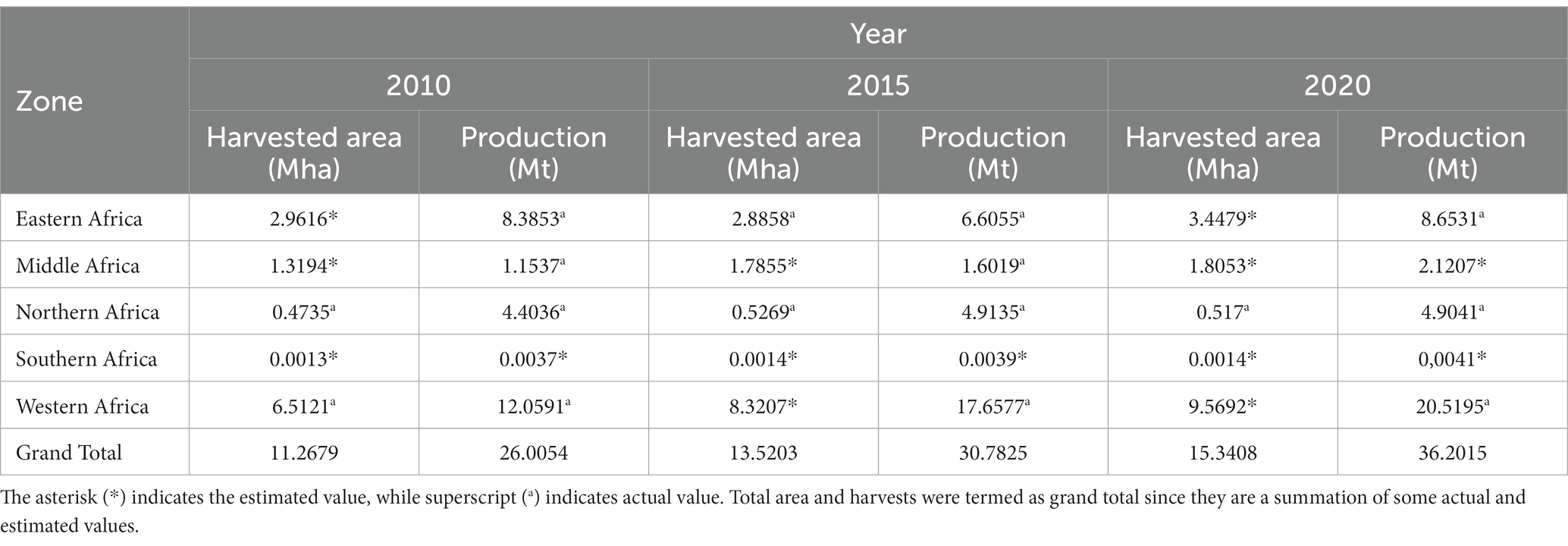
Table 1. Rice harvested area and production by African zones from 2010 to 2020 as per FAOSTAT Database (https://www.fao.org/faostat/en/#data/QCL; accessed February 20, 2023).
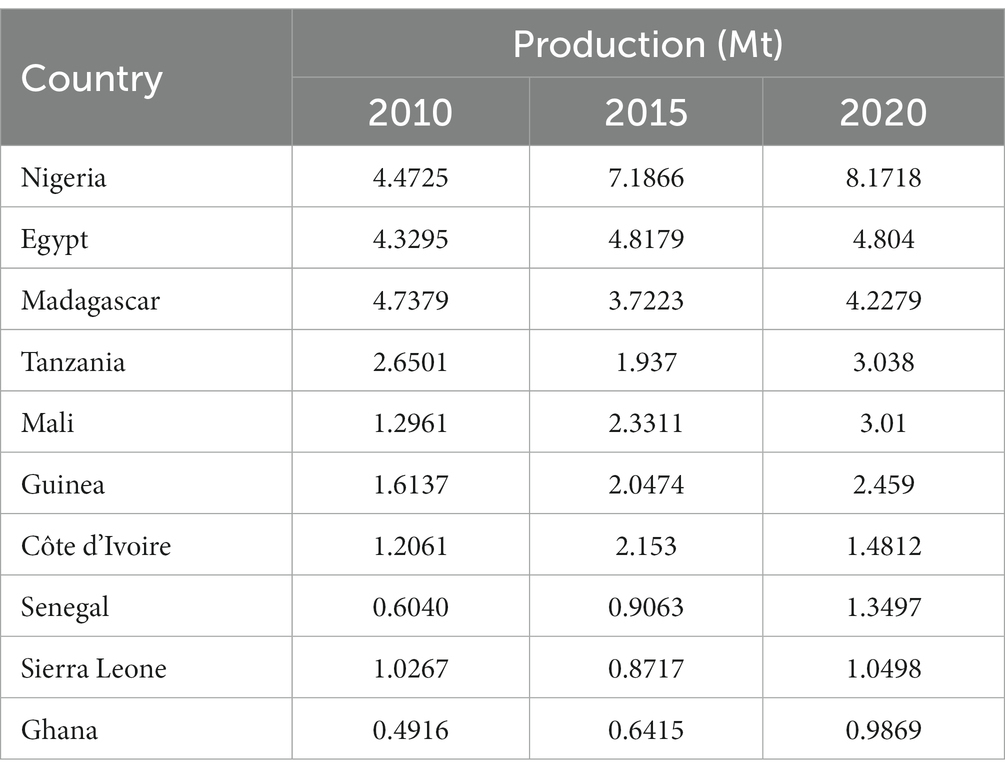
Table 2. Rice production statistics from 2010 to 2020 among the ten highest rice producers in Africa (FAOSTAT Database, https://www.fao.org/faostat/en/#data/QCL; accessed February 20, 2023).
Overall, the upward trend in rice production in recent years is primarily attributed to the expansion of rice cultivation areas, the introduction of rice varieties with desirable cooking traits and reduced shattering, productive research efforts such as the development of NERICA varieties, the involvement of farmers in varietal development, and increased awareness of good agricultural practices (Mogga et al., 2018; Arouna et al., 2021; Bin Rahman and Zhang, 2022).
2.3 Rice ecosystems
Africa’s rice ecosystems are diverse and vary across countries, with differing representations and proportions. Balasubramanian et al. (2007) categorized rice ecosystems in Africa into five groups: deep water and mangroves (9%), rainfed upland (38%), rainfed lowland (33%), and irrigated (20%). However, there have been slight variations in these proportions reported by different sources. Rodenburg and Johnson (2009) revised these figures with a slightly different distribution, reporting rainfed upland (39%), rainfed lowland (33%), and irrigated (19%), while deep water and mangroves covered only 9%. The presence of deep water and mangroves was noted in the flood plains of the Niger River, covering countries such as Guinea, Mali, and Nigeria, while coastal areas of Sierra Leone, Liberia, and Gambia had mangrove ecosystems.
Several other sources, including Africa Rice Center (AfricaRice) (2010), Sakagami and Kawano (2011), Sie et al. (2012), Diagne et al. (2013a), and Suvi et al. (2020), have reported similar rice ecosystems with minor variations in the distribution percentages. Despite these minor differences, the general trend remains consistent, with rainfed upland being the largest ecosystem, followed by rainfed lowland and irrigated. According to Africa Rice Center (AfricaRice) (2010), only 14% of the rice ecosystem is irrigated, while rainfed upland and lowland account for 40 and 37%, respectively. The distribution of these ecosystems can change over time due to shifts in land utilization patterns. Diagne et al. (2013a) reported a total rice cultivation area of 9.9 million hectares, with rainfed lowland (38%) being the most extensive ecosystem, followed by rainfed upland (32%) and irrigated (26%). In contrast, deep water and mangroves had the smallest share of rice cultivation area, with only 4% coverage. Generally, the distribution of rice ecosystems in Africa aligns with the findings of Diagne et al. (2013a) and Africa Rice Center (AfricaRice) (2019), which indicate that more than 70% of the rice area in Sub-Saharan Africa is rainfed. Among these ecosystems, rainfed upland, rainfed lowland, and irrigated are the most widespread and dominant (Diagne et al., 2013a; Rodenburg and Saito, 2022).
When it comes to rice production contribution, Africa Rice Center (AfricaRice) (2011) reported rainfed lowland as the largest contributor, accounting for 48% of total production, followed by irrigated (33%) and rainfed upland (19%). Productivity levels also vary among these ecosystems, with irrigated rice typically achieving higher yields compared to rainfed systems. According to Ibrahim et al. (2022), the average rice yield in Sub-Saharan Africa is around 2 t/ha, while well-utilized rainfed and irrigated systems can produce 4–9 t/ha and 8–11 t/ha, respectively. Africa Rice Center (AfricaRice) (2011) reported average yields of 1 t/ha for upland, 1–3 t/ha for lowland, and 3–6 t/ha for irrigated rice. However, these yields are still below the potential yields for each ecosystem, which are 2–4 t/ha, 3–6 t/ha, and 6–15 t/ha, respectively. Recent data published by Saito et al. (2019) indicated actual yields of 1.6 t/ha for rainfed upland, 2.6 t/ha for rainfed lowland, and 3.9 t/ha for irrigated rice. These values, while improved, still fall short of the ecosystems’ full potential yields.
2.4 Overall production, consumption, and imports
Over the years, there has been a persistent gap between rice production and demand in Africa. From 1961 to 2005, rice production grew at a rate of 3.23%, while demand outpaced it, increasing at a rate of 4.52% (Sie et al., 2012). Africa Rice Center (AfricaRice) (2011) also noted this trend and reported a deficit of 9.68 Mt. of milled rice, resulting in a cost of 5 billion USD for imports. This deficit persisted as the average rate of increase in rice production from 1970 to 2009 (3.3%) lagged behind the rate of consumption growth (4.0%). In recent years, there have been notable increases in rice production. For instance, in 2009, Seck et al. (2010) reported a production of 16 Mt. of milled rice, a significant milestone compared to the mere 2 Mt. produced in Sub-Saharan African countries in 1961. Zenna et al. (2017) compared rice production trends from 1992 to 2002 and 2003 to 2013, revealing an increase of 2 and 4%, respectively. This increase was attributed to the expansion of production areas, the use of improved varieties, and the adoption of modern agricultural inputs. Despite these production gains, rice imports have continued to rise. In 1961, only 0.5 Mt. of rice was imported into Africa from outside the continent (Seck et al., 2010). By 2003, this had grown to an imported rice cost of 1.5 billion USD (Balasubramanian et al., 2007). More recent statistics have also shown an increase in rice imports, primarily due to the rising consumption of rice among African nations (Rodenburg and Saito, 2022).
According to the FAO statistics for Crops and Livestock Products in 2023, total rice production in Africa reached 36.2 Mt. in 2020, up from 26 Mt. in 2010 and 30.8 Mt. in 2015. Despite this increase in production, rice imports have continued to grow. The annual report for 2016 by Africa Rice Center (AfricaRice) (2017) indicated that demand for rice in some African countries ranged from 10 to 90%, leading to an annual import cost of 5.5 billion USD to meet requirements. The consumption of rice in Africa has been on the rise. Tsujimoto et al. (2019) observed a significant increase in rice consumption from 9.2 Mt. in 1990 to 31.5 Mt. in 2019. In 2018, Africa spent almost 6 billion USD on importing nearly 25% of its rice for consumption. The average annual rice consumption from 2009 to 2019 was reported to be 27.4 Mt., while production lagged at only 15.4 Mt. (Ibrahim et al., 2022). Rodenburg and Saito (2022) noted that African rice imports accounted for almost 40% of the rice consumed, with the primary driver being the increasing demand for rice.
Projections for the future suggest that rice consumption will continue to rise. The FAO (2023b) projects a further increase in consumption to reach 34.9 Mt. in 2025. Nigatu et al. (2017) have also highlighted the risk of importing more rice, projecting an increase from 27 to 35 Mt. in 2026 if corrective measures are not implemented. These trends underscore the urgency of addressing the gap between rice production and consumption in Africa.
3 Rice production challenges and opportunities in Africa
3.1 Challenges
Diagne et al. (2013b) have categorized the challenges facing African rice farmers into various categories, including biotic, soil-related, and climate-induced factors. Africa Rice Center (AfricaRice) (2011) emphasizes that these factors are further exacerbated by socio-economic constraints. These challenges encompass a wide range, such as iron toxicity, droughts, floods, cold salinity, weed infestations, diseases, birds and rodent pests, suboptimal seed quality, impoverished soils, and inadequate post-harvest technologies (Balasubramanian et al., 2007; Rodenburg and Johnson, 2009; Diagne et al., 2013b; Van Oort, 2018; Hong et al., 2021).
The primary focus of this review is on flooding, a major challenge in both rainfed lowlands and irrigated areas. It is often triggered by unpredictable rains associated with climate change (Agbeleye et al., 2019). Farmers have recognized this stress, which ranks second only to drought in terms of its impact, accounting for a 27% yield loss across all ecological zones (Diagne et al., 2013b). The impacts of flooding are observed throughout lowland rice-growing areas, and in particularly stressful years, farmers experience total crop failure. This challenge manifests in various forms, affecting different stages of the rice production cycle, from sowing to harvest. Flooding affects germination, tillering, plant survival, lodging, spikelet fertility, grain weights, and ultimately yields. It also hinders the recovery of most rice cultivars (Panda and Barik, 2021).
In addition to flooding, other challenges significantly impact rice production in Africa. Drought, for instance, is a devastating impediment to rice production for African farmers. It resulted in a yield loss of 29% during the 2009–2010 period across all rice ecosystems, with upland rice being particularly susceptible (Diagne et al., 2013b). Moreover, weeds have consistently emerged as one of the most formidable biotic challenges, especially in rainfed rice farming, where yields can be less than 1 ton per hectare [Rodenburg and Johnson, 2009; Africa Rice Center (AfricaRice), 2011]. Parasitic weeds, including the Striga genus, inflict substantial monetary losses, estimated to range between 111 million and 200 million USD annually [Rodenburg and Johnson, 2009; Africa Rice Center (AfricaRice), 2017]. Other pests such as the African rice gall midge, birds, and rodents also pose significant threats to rice production. Furthermore, rice in Africa faces diseases such as bacterial leaf blight, Rice yellow mottle virus, and blast. Bacterial blight, for instance, inflicted yield losses ranging from 35 to 52% in Niger and 25% in the northern part of Benin [Africa Rice Center (AfricaRice), 2011; Suvi et al., 2020].
Mineral toxicity, particularly iron toxicity, has afflicted numerous African countries, leading to yield losses ranging from 10% to a staggering 100% (Melandri et al., 2021). West African nations have borne the brunt of the iron toxicity challenge, with nearly 60% of their cultivated areas affected (Sikirou et al., 2018). Likewise, the detrimental impact of excessive aluminium on rice crops has become increasingly apparent, affecting approximately 18.8% of rice-growing regions in Africa. Besides the biotic and abiotic challenges, the financial constraints and limited awareness among smallholder farmers pose significant barriers to their access to improved agricultural inputs and technologies. In addition to these challenges, many African soils suffer from nutrient deficiencies, particularly in nitrogen (N), phosphorus (P), potassium (K), and zinc (Zn) (Mohammed et al., 2014; Tsujimoto et al., 2019; Hong et al., 2021). It was found that more than 37.6% of rice-growing areas in Africa grapple with low soil nutrient levels, leading to reduced crop yields (Haefele et al., 2014). Furthermore, access to quality seeds and varieties represents another major challenge to sustainable rice farming in Africa, as highlighted in the Africa Rice Center’s annual report of 2010. Similarly, Futakuchi and Saito (2021) observed farmers’ overreliance on saved seeds for crop cultivation.
Finally, the post-harvest phase of rice production experiences losses in value, ranging between 15 and 50% (Somado et al., 2008). These losses result from suboptimal post-harvest practices, including the presence of impurities, chalkiness, heat damage, and a high quantity of broken rice [Africa Rice Center (AfricaRice), 2016]. Sub-Saharan Africa experiences post-harvest losses ranging from 9 to 17%, amounting to an estimated 14–600 million USD in losses each year [Africa Rice Center (AfricaRice), 2018]. Therefore, addressing these challenges is paramount for enhancing both the quality and quantity of rice production and, subsequently, improving overall food security in the region.
3.2 Unlocking Africa’s future potential for rice self-sufficiency
Africa’s rice production landscape stands as a realm of untapped potential, awaiting realization through a dedicated embrace of research and development endeavors aimed at fostering sustainable rice farming practices [Africa Rice Center (AfricaRice), 2011]. The age of heavy rice imports, which has placed undue pressure on Africa’s economic resources, must give way to a future where the continent takes charge of meeting its own rice demands. The sluggish pace of rice production growth in Africa, attributed to the underutilization of available arable land and persistently low productivity levels, calls for a strategic transformation (Ragasa and Chapoto, 2017; Rodenburg and Saito, 2022). Africa possesses the intrinsic potential to attain self-sufficiency once it commits to strategies that optimize land utilization and enhance overall productivity (Arouna et al., 2021).
Remarkably, Africa boasts vast expanses of land amenable to rice cultivation, yet only a fraction of its fertile wetlands currently witness the plow [Africa Rice Center (AfricaRice), 2011]. Astonishingly, out of the over 200 million hectares of wetlands spanning the African continent, a mere 5% find themselves under the cultivation of rice. It is this very underutilized land that, when accessed and cultivated, holds the power to significantly augment rice production. Furthermore, addressing the gap in the availability and accessibility of high-yielding rice varieties represents a pivotal opportunity for productivity enhancement [Africa Rice Center (AfricaRice), 2010]. Across the continent, farmers are predominantly cultivating traditional local rice varieties, relying on saved seeds year after year [Africa Rice Center (AfricaRice), 2012]. Yet beneath this challenge lies a wealth of diverse cultivars that remain ripe for improvement. Agricultural research institutions have been established in numerous countries, many with a particular focus on rice breeding. These initiatives promise to equip African farmers with superior quality and high-yielding rice varieties, consequently leading to an upsurge in output. Moreover, the rising demand for rice varieties tailored to withstand a gamut of stressors, from floods to prolonged dry spells, salinity, and nutrient imbalances, presents a compelling impetus for progress (Balasubramanian et al., 2007). The introduction of stress-tolerant rice varieties signifies more than just an expansion of cultivated land; it holds the promise of significantly enhancing productivity. Going beyond the NERICAs, the Africa Rice Center has successfully developed Sahel, ARICAs, and WITAs high-yielding varieties that are finely tuned to suit various African ecologies and withstand prevalent stresses. These varieties have the potential to bolster the livelihoods and incomes of smallholder farmers (Arouna et al., 2017). By making strategic investments in this endeavor, the continent stands poised to breathe new life into long-abandoned agricultural lands, ultimately resulting in a substantial increase in rice production.
To facilitate the rice transformative journey, the implementation of supportive agricultural policies takes center stage (FAO, 2023a). These policies wield the power to steer the trajectory of the rice sector’s development (Clapp, 2017). They are inextricably linked to initiatives such as mechanization, the subsidization of agricultural inputs, and the provision of accessible loans to farmers (Arouna et al., 2021). These measures collectively ensure the efficient organization of farming activities, the timely application of agronomic practices, and the seamless management of the post-harvest value chain. In addition, these policies are aligning with the national rice development strategies (NRDS), which are now being implemented by the majority of African countries. Therefore, Africa stands at the cusp of a remarkable transformation, one that holds the promise of self-sufficiency in rice production. By harnessing its abundant resources, embracing research and development, and fortifying the supportive policy landscape, the continent is poised to unlock its full potential and emerge as a beacon of sustainable rice farming.
4 Flooding and rice production in Africa
4.1 Weather variability and prevalence of floods
Flooding limits rice production, particularly in rainfed lowland ecosystems. This is exacerbated by the irregular and uncertain distribution of rainfall, in itself accelerated by climate change, which is a major concern today (Mackay, 2008). Weather variability has increased globally, and Africa’s heavy reliance on rainfed agriculture makes it highly vulnerable to weather extremes, including floods (Agbeleye et al., 2019). Projections for the future suggest an escalation in the frequency and intensity of weather extremes due to climate change [Atanga and Tankpa, 2021; World Meteorological Organization (WMO), 2022]. A report by the UN Office for the Coordination of Humanitarian Affairs (OCHA, 2022) identified 19 countries prone to floods with significant damage to farmlands. These countries include Chad, the Democratic Republic of Congo, Niger, Nigeria, Liberia, the Central African Republic, Gambia, Guinea, Mauritania, Senegal, Côte d’Ivoire, Ghana, Sierra Leone, Mali, Cameroon, Benin, and Burkina Faso. In 2021, approximately 15 African nations also experienced flooding. Akinyoade et al. (2014) conducted surveys in countries such as Kenya, Ethiopia, and Mozambique. The results revealed that more than 90% of respondents perceived the existence of floods and droughts as a major threat to crops.
Several countries such as Tanzania have a history of riverine flooding, especially in floodplains, which significantly affects overall agricultural productivity (Valimba and Mahe, 2020). Other countries, including Nigeria, Madagascar, Mali, Sierra Leone, Uganda, Burkina Faso, and Rwanda, have reported flood-related disruptions to agriculture in certain years [Africa Rice Center (AfricaRice), 2011, 2018; Anna et al., 2019]. The World Meteorological Organization (WMO) (2022) projected an increase in the severeness of climate extremes. Hence, the organization estimated that Africa will need to spend nearly $50 billion USD annually by 2050 to mitigate these weather-related losses. Therefore, addressing the challenges posed by flooding in rice production can never be neglected in Africa.
4.2 Types of flooding in rice
Ismail et al. (2012) identified four inundation stresses that rice farmers are likely to encounter: flooding at germination, flash flooding, long-term partial flooding, and deep water. They further distinguished these stresses, highlighting anaerobic germination, which encompasses submergence stress during germination caused by heavy rains occurring shortly after sowing. This phenomenon is prevalent among farmers who practice direct seeding. Flash floods are another perilous event that submerges crops for a short duration, typically one to two weeks, resulting in crop damage or death. This is distinct from long-term stagnant flooding, where water levels of 30–50 cm accumulate for a significant portion of the growing season. Stagnant flooding is sometimes referred to as partial flooding. Additionally, there are other types of flooding, such as deepwater and floating rice cultivation, where crops endure inundation up to several meters. Similar flooding stresses have been reported by researchers such as Bailey-Serres et al. (2010), Fukao et al. (2006), and Mackill et al. (2012).
Africa experiences similar types of flooding, consistent with global reports (Fukao et al., 2006; Bailey-Serres et al., 2010; Ismail et al., 2012; Mackill et al., 2012). Heavy rains can trigger flash floods, affecting both germination for directly seeded rice and submerging emerged seedlings for several weeks. Long-term water stagnation is another prevalent flooding type across African countries, characterized by the accumulation of water over an extended period during the rice-growing season, partially submerging crops (Agbeleye et al., 2019). Moreover, some coastal regions in Africa, such as Gambia and Guinea-Bissau, are prone to coastal floods, particularly in mangrove areas (Sakagami and Kawano, 2011). Additionally, there are regions where crops suffer from long-term complete submergence for over a month, as observed in areas cultivating deepwater and floating rice, notably in countries such as Chad, Mali, and Niger (Sakagami and Kawano, 2011).
4.3 Impact of floods on rice production and food security
Adverse impacts of floods have been observed all over Africa, posing significant losses to crop production. These impacts encompass failures in rice germination for flooded soils, crop losses resulting from flash floods, lodging of rice plants, and diminished vigor in cases of long-term stagnant flooding (Agbeleye et al., 2019). However, addressing these challenges in African rice farming is complicated by limited information and the scarcity of flood-tolerant rice varieties, leaving farmers highly vulnerable to climate extremes such as flooding (Akinyoade et al., 2014).
One region profoundly affected by flooding is Northern Ghana, where Atanga and Tankpa (2021) reported massive crop losses due to inundation, significantly affecting local food security. In Tanzania’s Kilombero floodplain, rice yields have dwindled to a mere 1 tonne per hectare, with water-control challenges cited as a contributing factor for this agricultural setback (Kwesiga et al., 2020). West Africa, too, has faced the harsh consequences of long-term complete submergence, resulting in severe crop losses (Kawano et al., 2009; Sakagami et al., 2009; Sakagami and Kawano, 2011). In Uganda, over 2,000 acres of rice fields suffered significant damage due to floods, resulting in substantial yield losses (Anna et al., 2019).
Africa Rice Center (AfricaRice) (2018) noted that approximately 22% of rice production losses in Nigeria in 2012 were attributed to floods, primarily linked to the use of susceptible rice cultivars. Burkina Faso experienced a similar challenge, with an estimated 50% of the irrigated rice ecosystem suffering inundation, while Rwanda encountered 40% yield losses due to comparable circumstances [Africa Rice Center (AfricaRice), 2011]. A survey conducted by the Africa Rice Center in 2009–2010 reflected farmers’ perceptions of flooding impacts, indicating an average yield loss of 27% across the rice ecosystem, with losses of 34, 27, and 25% in irrigated, rainfed lowland, and rainfed upland areas, respectively. Reed et al. (2022) reported that between 2009 and 2020, nearly 12% of people in Africa experienced food insecurity due to floods. Agbeleye et al. (2019) concurred that floods can result in losses ranging from 10 to 100%, depending on factors such as the rice cultivar., growth stage, flood duration, depth, and floodwater characteristics, as highlighted in Mackill et al. (2012). Akinyoade et al. (2014) found that only farmers using climate-resistant crop varieties and diversifying their crops were less sensitive to weather extremes.
Floods are recognized for inhibiting gaseous exchange in plants by a factor of 10,000 times and disrupting normal respiration, making them particularly lethal to crop plants (Fukao et al., 2006). This interference with the plant’s ability to respire and carry out essential metabolic functions leads to severe damage and even total crop losses, consequently causing acute food shortages.
5 Overcoming flooding impacts
5.1 Research and global remarkable success on flood-tolerant rice
5.1.1 Anaerobic germination for direct-seeded rice
Significant progress has been made in the identification and development of flood-tolerant rice varieties, as evidenced by a body of research (Mackill et al., 2012; Singh et al., 2017b; Kato et al., 2019; Mondal et al., 2020). This progress encompasses the characterization of morphological, physiological, molecular, and metabolic traits associated with flooding tolerance, as reported in the studies by Panda and Barik (2021) and Singh et al. (2017b). It is worth noting that most of these achievements have been concentrated in Asia as compared to other regions. In direct-seeded rice, the establishment of crops is particularly vulnerable to oxygen limitations associated with floods. Yamauchi et al. (1993) observed limited survival and uneven crop establishment when characterizing rice germination in flooded environments, even at relatively shallow depths of 2 to 5 cm. Under more stringent screening conditions, flooding at greater depths can lead to severe crop losses, as documented by Ismail et al. (2012).
Numerous studies have examined various rice accessions for variations in tolerance under oxygen-deprived conditions, with notable contributions from researchers such as Angaji et al. (2010), Baltazar et al. (2019), and Yang et al. (2022). High expression and increased activities of a key enzyme, ɑ-amylase, are crucial for ensuring the availability of sugars, which are essential for escaping stress (Ismail et al., 2009). It has been observed that cultivars capable of tolerating inundation during germination efficiently break down starch reserves to fuel the growing embryo and facilitate coleoptile access to air (Kretzschmar et al., 2015).
Furthermore, coleoptile elongation has been identified as a key factor determining rice survival in flooded soils and is now widely used in screening processes (Hsu and Tung, 2015; Zhang et al., 2017; Kuya et al., 2019; Pucciariello, 2020; Su et al., 2021; Thapa et al., 2022). Researchers have also identified the involvement of hormones, such as ethylene, specific enzymes such as ɑ-amylase, ADH, and PDC, and major QTLs such as AG1 and AG2 in flood tolerance. This understanding has led to the development of flood-tolerant, high-yielding rice varieties through the introgression of these QTLs, and these varieties have been introduced to farmers (Mondal et al., 2020). The introduction of these introgressed cultivars has significantly improved germination and yield compared to previously used susceptible cultivars.
5.1.2 Flash flooding tolerance
In the event of flash floods, farmers’ fields are completely submerged, which has a profound impact on crop survival and recovery (Septiningsih et al., 2009). Researchers have characterized Sub1, a quantitative trait locus (QTL) housing the Sub1A gene responsible for vegetative complete submergence tolerance (Xu and Mackill, 1996). Cultivars carrying the Sub1A1 allelic form of this gene were identified as capable of overcoming the stress and maintaining high underwater photosynthetic efficiency (Singh et al., 2020).
Studies have revealed that the primary mechanism associated with this tolerance is a quiescence strategy (Bailey-Serres et al., 2010; Pucciariello and Perata, 2013). Tolerant cultivars successfully withstand flash floods, displaying non-elongation, high survival rates, conservation of energy reserves, and improved post-submergence recovery (Fukao et al., 2006; Bailey-Serres et al., 2010; dos Santos et al., 2017). Additionally, Pedersen et al. (2009) emphasized the importance of gas films in enhancing submergence tolerance, with these micro-layers contributing to aeration even under stressful submergence conditions.
Notably, the Sub1 gene has been introgressed into popular mega varieties through marker-assisted backcrossing (Mackill et al., 2012). Consequently, farmers in Asia have been utilizing improved Sub1 varieties, including IR 64 Sub1, Swarna Sub1, Ciherang Sub1, Samba Mahsuri Sub1, and BR 11 Sub1, which have significantly enhanced yield under stress conditions.
5.1.3 Advances in stagnant flooding
Lowland areas often accumulate water for extended periods, partially submerging the crops, leading to a range of stressors affecting tillering, fertility, lodging, grain quality, and yield (Chattopadhyay et al., 2021). This challenge is particularly prevalent in low-lying fields prone to flooding. Some cultivars completely fail to survive during prolonged water stagnation.
In a study by Vergara et al. (2014), the response of 626 accessions partially submerged with 50–60 cm of water up to maturity was investigated. Stagnant flood-tolerant genotypes were characterized by moderate elongation, in contrast to semi-dwarf and fast-elongating accessions, which exhibited poor performance and low survival rates. Additionally, tolerant lines displayed minimal starch depletion compared to intolerant ones. Consequently, tolerance was attributed to factors such as moderate elongation, enhanced tillering capacity, reduced starch depletion, and increased fertility.
Singh et al. (2017a) reported the identification of 36 QTLs related to survival, growth traits, and yield under stagnant flooding conditions, primarily clustered on chromosomes 3 and 5. Chattopadhyay et al. (2021) identified 17 QTLs for partial submergence using a mapping population derived from a tolerant genotype, Rashpanjor, and Swarna, a widely grown high-yielding variety. Kato et al. (2019) highlighted IRRI119, IRRI154, and OR142-99 as released varieties with the ability to withstand stagnant flooding.
5.1.4 Deepwater-adapted cultivars
Internode elongation has been recognized as a dominant mechanism for overcoming deepwater stress (Hattori et al., 2011). Tolerant cultivars maintain increased internode elongation to stay in contact with air, in contrast to non-elongating rice types.
Since the discovery of the SNORKEL genes, SK1 and SK2, significant progress has been made in elucidating and confirming the mechanisms controlling tolerance (Hattori et al., 2009; Singh et al., 2017b). Additionally, the role of ethylene has been found to promote rapid internode elongation as a strategy for escaping water (Hattori et al., 2009). Importantly, once water recedes, deepwater-adapted cultivars keep their reproductive parts above the ground, a feature referred to as “kneeing ability” (Singh et al., 2017b; Nagai and Ashikari, 2023).
Tolerant cultivars exhibit rapid growth, with a significant increase in height, equivalent to up to 25 cm per day (Singh et al., 2017b). Some of the most frequently cited varieties with improved performance in deepwater conditions include C9285, Bhadua, and BRRI Dhan91 (Shalahuddin et al., 2020; Nagai and Ashikari, 2023). These varieties have been instrumental in enhancing the understanding of the mechanism of tolerance in deepwater and improving resilience through QTL mapping in breeding programs.
5.2 African progress on characterization and development of flood-tolerant rice
5.2.1 Anaerobic germination
Overreliance on direct seeding has exposed African farmers to the challenges of flooding caused by unpredictable rains (Kuya et al., 2019). The inundation of fields reduces oxygen availability to germinating seeds, hindering successful crop establishment and necessitating costly replanting. Despite these drawbacks, direct seeding remains a highly favored method due to its water-, labor-, and time-saving advantages (Darko Asante et al., 2021). In response to the anaerobic stress caused by flooding, various initiatives have been undertaken in Africa, including screening for stress-tolerant rice. In a study by Agbeleye et al. (2019), a screening of Oryza glaberrima identified five accessions with higher percent survival rates during anaerobic germination compared to the tolerant check variety, Khao Hlan On. These accessions are TOG 5980, TOG 5485, TOG 5505, TOG 16704, and TOG 8347. Currently, work is in progress to identify the QTLs associated with tolerance through bi-parental crosses, using these accessions and local sensitive cultivars.
Another screening conducted by Darko Asante et al. (2021) identified five genotypes capable of withstanding anaerobic stress. These genotypes, namely, OBOLO, ART68-12-1-1-B-B, ART64-31–1-1-B-B, CRI-1-21-5-12, and CRI-Enapa, displayed strong emergence from flooded conditions, with survival rates exceeding 75%. Presently, the Climate Smart African Rice Project, funded by DANIDA, is actively engaged in research aimed at developing flood and salinity-tolerant rice varieties. One of the project’s key components is the identification of donors with tolerance to anaerobic stress during germination. The most recent achievements under this project include the identification of ten potential donors exhibiting anaerobic stress tolerance during germination (Mwakyusa et al., 2023). These donors are as follows: Afaa Mwanza 1/159, IB126-Bug 2013A, Kanamalia, Kubwa Jinga, Magongo ya Wayungu, Mpaka wa Bibi, Mwangaza, Rojomena 271/10, Wahiwahi, and Tarabinzona. They were selected based on both phenotypic tolerance and genomic values. The donors hold significant potential for future work involving QTL mapping with bi-parental or multi-parental populations.
5.2.2 Short-term vegetative submergence
In cases where rice crops are completely submerged at the early stages of establishment, even for short durations, survival is compromised, and recovery is uncertain. Many lowland rice production areas in Africa are prone to frequent floods, which can devastate crop growth (Diagne et al., 2013b). One historically significant discovery in addressing this issue is the Sub1 QTL, a major genetic locus controlling tolerance to complete submergence (Xu and Mackill, 1996). This QTL facilitates tolerance by conserving energy as a survival response (dos Santos et al., 2017). Sub1 has shown promise and has been beneficial to African farmers in flood-prone rice-growing areas.
In Africa, a significant milestone was achieved through the Stress-Tolerant Rice for Africa and South Asia (STRASA) project, a collaboration between the International Rice Research Institute (IRRI) and the Africa Rice Center. This initiative employed marker-assisted backcrossing (MABC) to develop submergence-tolerant varieties integrated with high yields and preferred recurrent parents. The project deemed varieties successful only if they exhibited at least a 1-tonne per hectare yield gain under flooding stress compared to the recurrent parent, with no yield penalties under non-stress conditions. Notable varieties resulting from this project include FARO 66 and FARO 67, which have shown the ability to overcome short-term complete submergence.1 These varieties have become popular and are widely grown in West Africa.
In another study, Kawano et al. (2009) investigated a number of rice cultivars with African and Asian origins for their tolerance to 7 days of flash flooding. The conclusion was that African rice cultivars suffer from short-term complete submergence due to their elongation escape strategy, which leads to lodging and limited recovery post-de-submergence. Akinwale et al. (2012) evaluated 20 rice varieties completely submerged for two weeks and found that Sub1 mega varieties sourced from IRRI had higher survival rates and yields compared to non-Sub1 cultivars of African origin. Low survival and high yield reductions were observed for varieties with increased stem elongation, such as FARO 57 and FARO 52.
Anna et al. (2019) investigated cultivar responses to flash floods at the seedling stage in Uganda, revealing that none of the cultivars possessed the Sub1A-1 allele responsible for submergence tolerance. Most of the cultivars instead had Sub1A-2. Agbeleye et al. (2019) identified TOS 6454 as the best-suited accession out of 2002 Oryza glaberrima accessions screened for flooding tolerance. Although this accession did not surpass Swarna Sub1, a tolerant check, it significantly outperformed other accessions. This highlighted that most Oryza glaberrima varieties are not suited for short-term complete submergence but are more adapted to deepwater elongation. Recently, El Dessougi et al. (2022) screened 20 rice varieties from Sudan and South Sudan, including a known tolerant check, FR13A, for flash flooding tolerance. The results indicated nearly zero survival percentages for the cultivars, except for the tolerant check, which showed a 42.5% survival rate. Hence, ongoing research efforts remain essential to address the challenges faced by numerous African countries grappling with flooding stress.
5.2.3 Stagnant flooding
The prolonged accumulation of water in lowlands, resulting in the partial submergence of rice crops, is indeed a devastating issue. This condition makes the crops susceptible to lodging, which, in turn, affects growth, yield components, and overall productivity. Although this stress is prevalent in many rice-growing regions and significantly impacts yields, there is limited knowledge on how to effectively mitigate it. Agbeleye et al. (2019) conducted a screening of accessions for stagnant flooding and identified four accessions that exhibited higher grain yields (gm-2) compared to the best check, IRRI 119. These accessions were IG 48, Gervex 2,674, IG 133, and TOG 7148.
In a study by Sakagami et al. (2009), five cultivars, namely, Banjoulou, Nylon, IR71700-247-1-1-2, IR73020-19-2-B-3-2B, and Ye’le’1A, were used to investigate their response to partial submergence for a duration of 37 days at a water depth of 35 cm. Among these cultivars, only Ye’le’ represented Oryza glaberrima, while the rest were Oryza sativa. Ye’le’ is known for its strong escape response to deepwater conditions through elongation, but interestingly, it exhibited the lowest elongation in response to stagnant flooding.
Sakagami et al. (2013) reported that partially submerged rice cultivars experienced minimal growth effects compared to prolonged complete submergence. Another study by Oteyami et al. (2018) found that TOG 5810, an Oryza glaberrima variety, is well adapted to partial flooding. This cultivar displayed improvements in terms of a 1,000-grain weight while maintaining fewer empty grains per panicle. Currently, stagnant flooding is a significant concern addressed by the Climate Smart African Rice Project, which aims to develop rice varieties capable of withstanding water stagnation in lowland fields. This research is crucial for finding solutions to this challenging issue and improving rice production in flood-prone areas.
5.2.4 Deepwater rice
Prolonged flooding conditions, where rice crops are completely submerged, have devastating consequences for farmers. While this stress is most prevalent in some countries around the Niger Valley in Western Africa, total crop losses for less adapted rice varieties are a common occurrence. In some instances, water can accumulate to depths exceeding 1 meter for more than a month, hindering crop establishment and critical management practices. Interestingly, research and reports on this stress date back to the early 1900s, primarily focusing on countries such as Niger, Mali, Nigeria, Senegal, Gambia, Sierra Leone, Mauritania, and Benin (International Rice Research Institute, 1977). Local cultivars, mainly of the Oryza glaberrima species, were used by farmers but exhibited very low yields. In response to deepwater flooding, several West African countries invested in research. For example, in Mali, a number of Oryza sativa cultivars were introduced and tested in the 1960s, leading to the identification of varieties such as Malobadian, Indochina G, Nang Kiew, Khao Gaew, and Mali Sawn, which were best suited for deepwater conditions, with yields ranging from 3.7 to 4.5 t/ha, and were subsequently commercially released.
In Niger, a local variety called Demba Heira with an average yield of 3.6 t/ha was successfully appreciated and cultivated in deepwater. In the 1950s, Nigeria conducted evaluations of O. glaberrima for deepwater cultivation, identifying promising cultivars such as Badane, Tatan, Don Boto, and Farin Iri. In the 1960s, varieties such as Mali Ong, Godalaki, and Indochina Blanc were added to the list following intensive testing. The introduction and evaluations of O. sativa in Guinea and Sierra Leone resulted in the release of Indochina Blanc, which has been extensively cultivated in these two countries. Futakuchi et al. (2001) studied the response of African rice to submergence tolerance over a duration of three weeks. The study revealed that O. glaberrima exhibits greater tolerance and avoidance strategies through stem elongation compared to O. sativa when subjected to prolonged complete submergence. Sakagami et al. (2009) explored the physiological differences between Asian and African rice for long-term complete submergence. Seedlings were completely submerged for 31 days with a 50 cm water depth, including IRRI Sub1 checks. All O. glaberrima cultivars survived the stress with increased shoot elongation compared to Asian rice, while all Sub1 cultivars such as FR13A failed to survive. Cultivars such as Nylon and Ye’le’1A were confirmed to be adapted to deepwater following a similar trial involving submergence for 37 days with an 80 cm water level.
Furthermore, Sakagami et al. (2013) conducted another screening for complete submergence over a duration of 32 days. The findings showed an average survival rate of around 90% for African rice, while Asian rice cultivars exhibited survival rates of 40–50%. The Sub1 checks failed to survive the stress, displaying less than 5% survival. One outstanding survival case was displayed by CG14, an Oryza glaberrima cultivar., which showed 100% survival after 32 days of complete submergence and significant recovery. Strategies such as faster shoot elongation, anaerobic tillering, larger leaf area extension, higher photosynthetic rate, and the maintenance of PSII maximum efficiency are potentially used by O. glaberrima to survive prolonged deepwater conditions. Through the STASSA project, two cultivars, Kolondieba 2 and Kadia 24, were developed for lowland deepwater ecosystems. These cultivars were well distributed in Mali, covering farmers’ fields prone to long-term deep flooding, and have shown the ability to survive stress with improved yields (see footnote 2).
In a recent study, Luo et al. (2023) used African cultivated rice to study the mechanisms employed for survival in deepwater conditions. The findings indicated that internode elongation is highly expressed in deepwater-adapted cultivars. Additionally, genes promoting internode elongation, such as SNORKEL (SK1/SK2), SEMIDWARF1 (SD1), and ACCELERATOR OF INTERNODE ELONGATION 1 (ACE1), were characterized by increased internode elongation in the presence of SK genes. Among the allelic forms of the SK2 gene, SK2-B was found to be highly significant in strongly accelerating internode elongation during flooding. Cultivars such as CG14, C8992, RAM3, C8872, C8991, C8892, and W0844 displayed the highest internode elongation and can hence be considered useful resources for variety development.
5.3 Potential advantages of flood-tolerant rice in Africa
Agbeleye et al. (2019) highlighted WITA 4 as an outstanding variety, surpassing the known checks IRRI 119 and IRRI 154 in both survival and yields. In stagnant flooding conditions at a 50 cm water depth for three months, WITA 4 exhibited an impressive survival rate of 78%. Most notably, the variety experienced only a 4% reduction in yield compared to the control conditions. Under stress conditions, it yielded 385 g/m2, equivalent to 3.85 t/ha, while IRRI 119 and IRRI 154 yielded only 220 and 144 g/m2, respectively. Therefore, the adoption of this variety for cultivation and as a donor is of significant importance and has the potential to bring about a revolution in fields prone to stagnant flooding.
Africa Rice Center (AfricaRice) (2020) reported its results of the evaluation of FARO 66 and 67, which were released in Nigeria in 2017. These flood-tolerant varieties were derived from the Sub1-introgressed mega varieties WITA 4 and NERICA-L 19 through marker-assisted breeding. The average yield advantages ranged from 10 to 80 times during submergence and a 6–29% higher yield when there was no stress compared to their recurrent parents. The UNDRR (2017) highlighted that FARO 66 was superior to its recurrent parent WITA 4 (FARO 52); hence, under submergence, it yielded 80 times, and under non-submergence, it had a yield gain of 6–11%. FARO 67 yielded 10 times under submergence, while under non-submergence, it had a 10–29% yield advantage compared to its recurrent parent NERICA- L19 (FARO 60).
Another study by Ulzen et al. (2022) compared the performance of improved varieties to lowland ecology, among which FARO 66 and 67 were included. The farmers selected FARO 66 as their preferred variety over the others due to its high yield and large panicles. The comparison of varietal resilience to submergence when wet seeded or transplanted using FARO 66, FARO 67, and a local check WITA 9 in Cote d′Ivoire indicated that the yields of two submergence varieties (FARO 66 and FARO 67) are not affected by either the growing season or crop establishment method unlike the local check (Devkota et al., 2022). The yields of the submergence tolerant varieties, regardless of the growing season and establishment method, were 1.1–4.5 t/ha higher than those of WITA 9. FARO 66 and FARO 67 were declared among the Sub1 climate-resilient variety with a yield advantage of 1–3 t/ha over the original varieties (CGIAR, 2021). Therefore, with such yield advantages, farmers adopting these varieties are more likely to be successful and overcome food scarcity during harsh weather.
5.4 Learning from the experiences in Asia
Numerous studies conducted in Asia have provided valuable insights into the mechanisms of stress tolerance in rice and the subsequent development of tolerant rice varieties. This wealth of research has made Asia a prime example from which valuable lessons can be drawn regarding how farmers have benefited from the adoption of stress-tolerant rice varieties. These studies have demonstrated the positive impact of utilizing such varieties. Mondal et al. (2020) conducted an evaluation of 10 cultivars for anaerobic germination. These cultivars were subjected to dry direct seeding, and seeds were submerged with a water depth of 3–5 cm for 21 days. Their findings revealed that the popular varieties with anaerobic germination QTLs AG1 and AG2 demonstrated a significantly higher yield of 2.8 t/ha compared to their counterparts lacking these QTLs. Furthermore, the varieties introgressed with AG QTLs exhibited a greater number of tillers, indicating their potential for improved rice production.
Similarly, Mackill et al. (2012) reported the results of multisite evaluations of Sub1 varieties paired with their non-Sub1 counterparts. These evaluations involved subjecting the rice to complete submergence for more than 5 days. Notably, Swarna Sub1 and Samba Mahsuri Sub1 yielded 3.67 and 3.8 t/ha, respectively, in contrast to their non-Sub1 counterparts, which yielded 2.34 and 2.1 t/ha. The Sub1 varieties exhibited yields that were twice as high during extended periods of submergence compared to the non-Sub1 varieties. Furthermore, submergence-tolerant cultivars exhibited characteristics such as greater chlorophyll retention and improved seedling recovery.
Moreover, farmers in countries such as India, Bangladesh, Nepal, the Philippines, and Myanmar have adopted Sub1 rice varieties. These include popular varieties such as Swarna Sub1, IR64 Sub1, BR11 Sub1, and Samba Mahsuri Sub1. The farmers assessed various traits, including survival, recovery, tillering, plant height, panicle length, grain color, length, and quality, post-harvest qualities, and overall yields. The Sub1 varieties consistently outperformed locally used cultivars in the criteria assessed by the farmers. This not only contributed to improved overall productivity but also had a positive impact on food availability and living standards.
6 Conclusion
This review has examined the progress in Africa’s development of flood-tolerant rice varieties. While some strides have been made, the region’s achievements remain limited, underscoring the critical importance of flood-tolerant rice varieties for improving productivity and ensuring food security. The scattered nature of information on developing flood-tolerant rice varieties highlights the need for effective coordination and thorough documentation. Despite the limited number of flood stress-tolerant varieties currently available in the region, it is imperative to ensure their widespread dissemination in flood-prone rice cultivation areas. This requires studying farmers’ perceptions, adoption rates, and the performance of these varieties as data on these aspects are scarce. Such studies can provide valuable insights into crop survival, quality, and overall performance, potentially leading to significant improvements in farmers’ harvests and food availability. Nonetheless, it is essential to recognize that further efforts are still needed in the identification and development of flood stress-tolerant varieties, given the alarming intensity and frequency of floods in Africa. Furthermore, there is also a crucial need for the development of rice varieties that can withstand multiple types of flooding. Currently, no such varieties are available, even though the same ecological regions can experience diverse types of flooding. Therefore, the future success of Africa’s rice sector hinges on dedicated and productive research efforts, coupled with effective dissemination and adoption strategies.
Author contributions
LM designed the study concept, conducted the literature review, and drafted the manuscript. SD, MH, MCH, RM, and NK edited the study concept and reviewed the manuscript. All authors contributed to the article and approved the submitted version.
Funding
This study was funded by DANIDA through the Climate Smart African Rice Research Project with Grant No. 18-03-KU.
Acknowledgments
The authors greatly acknowledge the Climate Smart African Rice Research Project for funding this study.
Conflict of interest
The authors declare that the research was conducted in the absence of any commercial or financial relationships that could be construed as a potential conflict of interest.
Publisher’s note
All claims expressed in this article are solely those of the authors and do not necessarily represent those of their affiliated organizations, or those of the publisher, the editors and the reviewers. Any product that may be evaluated in this article, or claim that may be made by its manufacturer, is not guaranteed or endorsed by the publisher.
Footnotes
1. ^Source: https://strasa.irri.org/home.
References
Africa Rice Center (AfricaRice). (2010). Africa Rice Center (AfricaRice) annual report 2009: Increasing investment in Africa’s rice sector. AfricaRice: Cotonou, Benin: 68 pp.
Africa Rice Center (AfricaRice). (2011). Africa Rice Center (AfricaRice) annual report 2010: Building African capacity on policy analysis and impact assessment. AfricaRice, Cotonou, Benin: 80 pp.
Africa Rice Center (AfricaRice). (2012). Africa Rice Center (AfricaRice) annual report 2011: A new rice research for development strategy for Africa. AfricaRice, Cotonou, Benin: 96 pp.
Africa Rice Center (AfricaRice). (2016). Africa Rice Center (AfricaRice) annual report 2015: Investing in rice research and innovation for Africa. AfricaRice: Abidjan, Côte d’Ivoire: 32 pp.
Africa Rice Center (AfricaRice). (2017). Africa rice Center (AfricaRice) annual report 2016: Towards rice self-sufficiency in Africa. AfricaRice: Abidjan, Côte d’Ivoire: 36 pp.
Africa Rice Center (AfricaRice). (2018). Africa Rice Center (AfricaRice) annual report 2017: More effective targeting of research for development. AfricaRice: Abidjan, Côte d’Ivoire: 40 pp.
Africa Rice Center (AfricaRice). (2019). Africa Rice Center (Africa Rice) annual report 2018: Sustainable rice production in the face of climate emergency. AfricaRice: Abidjan, Côte d’Ivoire: 28 pp.
Africa Rice Center (AfricaRice). (2020). Africa Rice Center (AfricaRice) annual report 2019: Toward rice-based foodsystems transformation in Africa. AfricaRice: Abidjan, Côte d’Ivoire: 28 pp.
Agbeleye, O. A., Olubiyi, M. R., Ehirim, B. O., Shittu, A. O., Jolayemi, O. L., Adetimirin, V. O., et al. (2019). Screening African Rice (O. glaberrima Steud.) for tolerance to abiotic stress. III. Flooding. SABRAO J. Breed. Genet. 51, 128–150.
Akinwale, M. G., Akinyele, B. O., Odiyi, A. C., Nwilene, F., Gregorio, G., and Oyetunji, O. E. (2012). Phenotypic screening of Nigerian rainfed lowland mega Rice varieties for submergence tolerance. Proc. World Congress Eng. 1, 4–6.
Akinyoade, A., Klaver, W., Soeters, S., and Foeken, D. (2014). Digging deeper: Inside Africa’s agricultural, Food and Nutrition Dynamics. Brill. doi: 10.1163/9789004282698
Angaji, S. A., Septiningsih, E. M., Mackill, D. J., and Ismail, A. M. (2010). QTLs associated with tolerance of flooding during germination in rice (Oryza sativa L.). Euphytica 172, 159–168. doi: 10.1007/s10681-009-0014-5
Anna, B. M., Paul, G., Richard, E., Jean, C. H., Gerald, M., Jimmy, L., et al. (2019). Identification of rice genotypes tolerant to submergence at seedling stage in Uganda. J. Plant Breed. Crop Sci. 11, 173–184. doi: 10.5897/jpbcs2019.0827
Arouna, A., Devkota, K. P., Yergo, W. G., Saito, K., Frimpong, B. N., Adegbola, P. Y., et al. (2021). Assessing rice production sustainability performance indicators and their gaps in twelve sub-Saharan African countries. Field Crop Res. 271, 108263–108216. doi: 10.1016/j.fcr.2021.108263
Arouna, A., Lokossou, J. C., Wopereis, M. C. S., Bruce-Oliver, S., and Roy-Macauley, H. (2017). Contribution of improved rice varieties to poverty reduction and food security in sub-Saharan Africa. Glob. Food Sec. 14, 54–60. doi: 10.1016/j.gfs.2017.03.001
Atanga, R. A., and Tankpa, V. (2021). Climate change, flood disaster risk and food security nexus in northern Ghana. Front. Sustain. Food Syst. 5, 1–11. doi: 10.3389/fsufs.2021.706721
Bailey-Serres, J., Fukao, T., Ronald, P., Ismail, A., Heuer, S., and Mackill, D. (2010). Submergence tolerant rice: SUB1’s journey from landrace to modern cultivar. Rice 3, 138–147. doi: 10.1007/s12284-010-9048-5
Bairagi, S., Bhandari, H., Kumar Das, S., and Mohanty, S. (2021). Flood-tolerant rice improves climate resilience, profitability, and household consumption in Bangladesh. Food Policy 105:102183. doi: 10.1016/j.foodpol.2021.102183
Balasubramanian, V., Sie, M., Hijmans, R. J., and Otsuka, K. (2007). Increasing rice production in sub-Saharan Africa: challenges and opportunities. Adv. Agron. 94:4002. doi: 10.1016/s0065-2113(06)94002-4
Baltazar, M. D., Ignacio, J. C. I., Thomson, M. J., Ismail, A. M., Mendioro, M. S., and Septiningsih, E. M. (2019). QTL mapping for tolerance to anaerobic germination in rice from IR64 and the aus landrace Kharsu 80A. Breed. Sci. 69, 227–233. doi: 10.1270/jsbbs.18159
Bin Rahman, A. N., and Zhang, J. (2022). Trends in rice research: 2030 and beyond. Food Energy Security 12:390. doi: 10.1002/fes3.390
CGIAR. (2021). Climate-smart rice. Available at: https://www.cgiar.org/innovations/climate-smart-rice/
Chattopadhyay, K., Chakraborty, K., Samal, P., and Sarkar, R. K. (2021). Identification of QTLs for stagnant flooding tolerance in rice employing genotyping by sequencing of a RIL population derived from Swarna × Rashpanjor. Physiology and molecular biology of plants. Int. J. Funct. Plant Biol. 27, 2893–2909. doi: 10.1007/s12298-021-01107-x
Chemutai, L. R., Musyoki, M. A., Kioko, W. F., Mwenda, N. G., Muriira, S., and Piero, N. M. (2016). Genetic diversity studies on selected rice (Oryza sativa L.) genotypes based on gel consistency and alkali digestion. Rice Res. 4, 1–6. doi: 10.4172/2375-4338.1000172
Clapp, J. (2017). Food self-sufficiency: making sense of it, and when it makes sense. Food Policy 66, 88–96. doi: 10.1016/j.foodpol.2016.12.001
Darko Asante, M., Ipinyomi, S. O., Abe, A., Adjah, K. L., Aculey, P., Kwame Bam, R., et al. (2021). Genetic variability for and tolerance to anaerobic germination in rice (Oryza sativa L.). J. Crop Improv. 35, 832–847. doi: 10.1080/15427528.2021.1884631
Devkota, K. P., Futakuchi, K., Mel, V. C., and Humphreys, E. (2022). Does wet seeding combined with SUB1 varieties increase yield in submergence prone lowlands of West Africa? Field Crop Res. 276:108375. doi: 10.1016/j.fcr.2021.108375
Diagne, A., Alia, D. Y., Amovin-Assagba, E., Wopereis, M. C., Saito, K., and Nakelse, T. (2013b). Farmer perceptions of the biophysical constraints to rice production in sub-Saharan Africa, and potential impact of research. Realizing Africa’s Rice Promise, 46–68. doi: 10.1079/9781845938123.0046
Diagne, A., Amovin-Assagba, E., Futakuchi, K., and Wopereis, M. C. (2013a). Estimation of cultivated area, number of farming households and yield for major rice-growing environments in Africa. Realizing Africa’s Rice Promise, 35–45. doi: 10.1079/9781845938123.0035
dos Santos, R. S., da Farias, D., Pegoraro, C., Rombaldi, C. V., Fukao, T., Wing, R. A., et al. (2017). Evolutionary analysis of the SUB1 locus across the Oryza genomes. Rice 10, 4–5. doi: 10.1186/s12284-016-0140-3
Dramé, K. N., Manneh, B., and Ismail, A. M. (2013). Rice genetic improvement for abiotic stress tolerance in Africa. Realizing Africa’s Rice Promise, 144–160. doi: 10.1079/9781845938123.0144
El Dessougi, H., Gasim, S., Abuanja, I., Abdella, A., and Nile, E. (2022). Screening for complete submergence seedling tolerance in Rice (Oryza sativa L.) genotypes collected from Sudan and South Sudan. African J. Plant Sci. 16, 250–257. doi: 10.5897/AJPS2022.2270
FAO. (2023a). Workshop report: exchange of experience on successful rice sector development policies in sub-Saharan Africa and Asia – Dar Es Salaam, United Republic of Tanzania, 24–26 October 2022. Accra. Available at: https://doi.org/10.4060/cc6203en
FAO. (2023b). Growing demand for rice sees Africa turn to experience sharing opportunities with Asia. Available at: https://www.fao.org/africa/news/detail-news/en/c/1612346/ (Accessed February 19, 2023)
FAOSTAT. (2012). Food and agriculture Organization of the United Nations Data. Statistical Databases. Available at: http://faostat.fao.org/default.aspx?lang=en
FAOSTAT. (2023). Statistics for crops and livestock products. Available at: https://www.fao.org/faostat/en/#data/QCL (Accessed February 19, 2023)
Fukagawa, N. K., and Ziska, L. H. (2019). Rice: importance for global nutrition. J. Nutr. Sci. Vitaminol. 65, S2–S3. doi: 10.3177/jnsv.65.S2
Fukao, T., Xu, K., Ronald, P. C., and Bailey-Serres, J. (2006). A variable cluster of ethylene response factor-like genes regulates metabolic and developmental acclimation responses to submergence in rice. Plant Cell 18, 2021–2034. doi: 10.1105/tpc.106.043000
Futakuchi, K., Jones, M. P., and Ishii, R. (2001). Physiological and morphological mechanisms of submergence resistance in African Rice (Oryza glaberrima Steud.). Japan. Soc. Trop. Agric. 45, 8–14.
Futakuchi, K., and Saito, K. (2021). Integrated research for development approach to upgrade the whole rice value-chain in sub-Saharan Africa: contribution of Africa Rice Center after NERICA (new rice for Africa). Japan Agricult. Res. Quarter. 55, 473–481. doi: 10.6090/jarq.55.473
Haefele, S. M., Nelson, A., and Hijmans, R. J. (2014). Soil quality and constraints in global rice production. Geoderma 235-236, 250–259. doi: 10.1016/j.geoderma.2014.07.019
Hattori, Y., Nagai, K., and Ashikari, M. (2011). Rice growth adapting to Deepwater. Curr. Opin. Plant Biol. 14, 100–105. doi: 10.1016/j.pbi.2010.09.008
Hattori, Y., Nagai, K., Furukawa, S., Song, X. J., Kawano, R., Sakakibara, H., et al. (2009). The ethylene response factors SNORKEL1 and SNORKEL2 allow rice to adapt to deep water. Nature 460, 1026–1030. doi: 10.1038/nature08258
Hong, S., Hwang, S., Lamo, J., Nampamya, D., and Park, T.-S. (2021). The current status of opportunities for rice cultivation in Uganda. J. Korean Soc. Int. Agric. 33, 67–74. doi: 10.12719/ksia.2021.33.1.67
Hsu, S., and Tung, C. (2015). Genetic mapping of anaerobic germination-associated QTLs controlling coleoptile elongation in rice. Rice 8, 38–12. doi: 10.1186/s12284-015-0072-3
Ibrahim, A., Saito, K., Kokou, A., Johnson, J.-M., Diagne, M., Fagnombo, D. J., et al. (2022). Seizing opportunity towards sustainable rice cultivation in sub-Saharan Africa. Environ. Sustain. Indicators 15:100189. doi: 10.1016/j.indic.2022.100189
International Rice Research Institute. (1977). Proceedings, 1976 deep-water Rice workshop, 8–10 November, Bangkok, Thailand. International Rice Research Institute: Los Baños, Philippines.
Ismail, A. M., Ella, E. S., Vergara, G. V., and Mackill, D. J. (2009). Mechanisms associated with tolerance to flooding during germination and early seedling growth in rice (Oryza sativa). Ann. Bot. 103, 197–209. doi: 10.1093/aob/mcn211
Ismail, A. M., Johnson, D. E., Ella, E. S., Vergara, G. V., and Baltazar, A. M. (2012). Adaptation to flooding during emergence and seedling growth in rice and weeds, and implications for crop establishment. AoB Plants 2012, 1–18. doi: 10.1093/aobpla/pls019
Kato, Y., Collard, B. C., Septiningsih, E. M., and Ismail, A. M. (2019). Increasing flooding tolerance in rice: combining tolerance of submergence and of stagnant flooding. Ann. Bot. 124, 1199–1209. doi: 10.1093/aob/mcz118
Kawano, N., Ito, O., and Sakagami, J.-I. (2009). Morphological and physiological responses of rice seedlings to complete submergence (flash flooding). Ann. Bot. 103, 161–169. doi: 10.1093/aob/mcn171
Kretzschmar, T., Pelayo, M. A. F., Trijatmiko, K. R., Gabunada, L. F. M., Alam, R., Jimenez, R., et al. (2015). A trehalose-6-phosphate phosphatase enhances anaerobic germination tolerance in rice. Nat. Plants 1:15124. doi: 10.1038/nplants.2015.124
Kuya, N., Sun, J., Iijima, K., Venuprasad, R., and Yamamoto, T. (2019). Novel method for evaluation of anaerobic germination in rice and its application to diverse genetic collections. Breed. Sci. 69, 633–639. doi: 10.1270/jsbbs.19003
Kwesiga, J., Grotelüschen, K., Senthilkumar, K., Neuhoff, D., Döring, T. F., and Becker, M. (2020). Rice yield gaps in smallholder systems of the Kilombero floodplain in Tanzania. Agronomy 10:1135. doi: 10.3390/agronomy10081135
Longtau, S. R. (2003). A review and description of rice production systems in Nigeria. Multi-agency partnerships in west African agriculture. Eco-systems Development Organisation: Jos, Nigeria.
Luo, Q., Sasayama, D., Nakazawa, M., Hatanaka, T., Fukayama, H., and Azuma, T. (2023). Deepwater response in the African cultivated rice Oryza glaberrima. Plant Produc. Sci. 26, 65–75. doi: 10.1080/1343943x.2022.2161401
Mackay, A. (2008). Climate change 2007: impacts, adaptation and vulnerability. Contribution of working group II to the fourth assessment report of the intergovernmental panel on climate change. J. Environ. Qual. 37:2407. doi: 10.2134/jeq2008.0015br
Mackill, D. J., Ismail, A. M., Singh, U. S., Labios, R. V., and Paris, T. R. (2012). Development and rapid adoption of submergence-tolerant (Sub1) Rice varieties. Adv. Agron. 115, 299–352. doi: 10.1016/B978-0-12-394276-0.00006-8
Melandri, G., Sikirou, M., Arbelaez, J. D., Shittu, A., Semwal, V. K., Konaté, K. A., et al. (2021). Multiple small-effect alleles of indica origin enhance high iron-associated stress tolerance in rice under field conditions in West Africa. Front. Plant Sci. 11, 1–21. doi: 10.3389/fpls.2020.604938
Mogga, M., Sibiya, J., Shimelis, H., Lamo, J., and Ochanda, N. (2018). Appraisal of major determinants of rice production and farmers’ choice of rice ideotypes in South Sudan: implications for breeding and policy interventions. Exp. Agric. 55, 143–156. doi: 10.1017/s0014479718000017
Mohammed, U. A., Abubakar, Z. A., Jibo, A. U., Abdullahi, M. B., and Abdul Hameed, A. (2014). Response of some rice cultivars to abiotic stress. Int. J. Agric. Sci. 4, 312–317.
Mondal, S., Khan, M. I., Dixit, S., Sta Cruz, P. C., Septiningsih, E. M., and Ismail, A. M. (2020). Growth, productivity and grain quality of AG1 and AG2 qtls introgression lines under flooding in direct-seeded Rice system. Field Crop Res. 248, 107713–107712. doi: 10.1016/j.fcr.2019.107713
Muthayya, S., Sugimoto, J. D., Montgomery, S., and Maberly, G. F. (2014). An overview of global rice production, supply, trade, and consumption. Ann. N. Y. Acad. Sci. 1324, 7–14. doi: 10.1111/nyas.12540
Mutiga, S. K., Rotich, F., Were, V. M., Kimani, J. M., Mwongera, D. T., Mgonja, E., et al. (2021). Integrated strategies for durable rice blast resistance in sub-Saharan Africa. Plant Dis. 105, 2749–2770. doi: 10.1094/pdis-03-21-0593-fe
Mwakyusa, L., Heredia, M. C., Kilasi, N. L., Madege, R. R., Herzog, M., and Dixit, S. (2023). Screening of potential donors for anaerobic stress tolerance during germination in Rice. Front. Plant Sci. 14, 1–13. doi: 10.3389/fpls.2023.1261101
Nagai, K., and Ashikari, M. (2023). Molecular mechanism of internode elongation in rice. Breed. Sci. 73, 108–116. doi: 10.1270/jsbbs.22086
Nigatu, G., Hansen, J., Childs, N., and Seeley, R. (2017). Sub-Saharan Africa is projected to be the leader in global rice imports. USDA-ERS, Washington, DC. Available at: https://www.ers.usda.gov/amber-waves/2017/october/sub-saharan-africa-is-projected-to-be-the-leader-in-global-rice-imports
OCHA. (2022). West and Central Africa: Flooding situation. Available at: https://reliefweb.int/report/mauritania/west-and-central-africa-flooding-situation-9-september-2022 (Accessed February 23, 2023)
Oteyami, M., Codjia, E., Agbangla, C., Ahoton, L., Santos, C., Montcho, D., et al. (2018). Submergence tolerance in irrigated and rainfed lowland Rice varieties: Agro-morphological basis for Rice genetic improvement in southern Benin. Open Access Library J. 5:e4304, 1–15. doi: 10.4236/oalib.1104304
Panda, D., and Barik, J. (2021). Flooding tolerance in Rice: focus on mechanisms and approaches. Rice Sci. 28, 43–57. doi: 10.1016/j.rsci.2020.11.006
Pedersen, O., Rich, S. M., and Colmer, T. D. (2009). Surviving floods: leaf gas films improve O₂ and CO₂ exchange, root aeration, and growth of completely submerged rice. Plant J. 58, 147–156. doi: 10.1111/j.1365-313X.2008.03769.x
Pucciariello, C. (2020). Molecular mechanisms supporting rice germination and coleoptile elongation under low oxygen. Plan. Theory 9, 1–11. doi: 10.3390/plants9081037
Pucciariello, C., and Perata, P. (2013). Quiescence in rice submergence tolerance: an evolutionary hypothesis. Trends Plant Sci. 18, 377–381. doi: 10.1016/j.tplants.2013.04.007
Ragasa, C., and Chapoto, A. (2017). Limits to green revolution in Rice in Africa: the case of Ghana. Land Use Policy 66, 304–321. doi: 10.1016/j.landusepol.2017.04.052
Reed, C., Anderson, W., Kruczkiewicz, A., Nakamura, J., Gallo, D., Seager, R., et al. (2022). The impact of flooding on food security across Africa. Proc. Natl. Acad. Sci. 119:e2119399119. doi: 10.1073/pnas.2119399119
Rodenburg, J., and Johnson, D. E. (2009). Weed management in rice-based cropping systems in Africa. Adv. Agron. 103:3004. doi: 10.1016/s0065-2113(09)03004-1
Rodenburg, J., and Saito, K. (2022). Towards sustainable productivity enhancement of rice-based farming systems in sub-Saharan Africa. Field Crop Res. 287:108670. doi: 10.1016/j.fcr.2022.108670
Roy-Macauley, H. (2018). Trends in Rice production and consumption during the CARD period 2008–2018. Paper presented at the 7th general meeting of the Coalition for African Rice Development, Tokyo, Japan, 2–4 October 2018.
Saito, K., Vandamme, E., Johnson, J.-M., Tanaka, A., Senthilkumar, K., Dieng, I., et al. (2019). Yield-limiting macronutrients for rice in sub-Saharan Africa. Geoderma 338, 546–554. doi: 10.1016/j.geoderma.2018.11.036
Sakagami, J.-I., Joho, Y., and Ito, O. (2009). Contrasting physiological responses by cultivars of Oryza sativa and O. glaberrima to prolonged submergence. Ann. Bot. 103, 171–180. doi: 10.1093/aob/mcn201
Sakagami, J.-I., Joho, Y., and Sone, C. (2013). Complete submergence escape with shoot elongation ability by underwater photosynthesis in African rice, Oryza glaberrima Steud. Field Crop Res. 152, 17–26. doi: 10.1016/j.fcr.2012.12.015
Sakagami, J.-I., and Kawano, N. (2011). Survival of submerged rice in a flood-prone region of West Africa. Tropics 20, 55–66. doi: 10.3759/tropics.20.55
Seck, P. A., Tollens, E., Wopereis, M. C. S., Diagne, A., and Bamba, I. (2010). Rising trends and variability of rice prices: threats and opportunities for sub-Saharan Africa. Food Policy 35, 403–411. doi: 10.1016/j.foodpol.2010.05.003
Septiningsih, E. M., Pamplona, A. M., Sanchez, D. L., Neeraja, C. N., Vergara, G. V., Heuer, S., et al. (2009). Development of submergence-tolerant rice cultivars: the Sub1 locus and beyond. Ann. Bot. 103, 151–160. doi: 10.1093/aob/mcn206
Shalahuddin, A. K., Masuduzzaman, A. S., Ahmed, M. M., Aditya, T. L., and Kabir, M. S. (2020). Development of high yielding deep water rice variety BRRI dhan91 for semi deep flooded ecosystem of Bangladesh. Bangladesh Rice J. 23, 57–63. doi: 10.3329/brj.v23i1.46081
Sie, M., Sanni, K., Futakuchi, K., Manneh, B., Mande, S., Vodouhe, R., et al. (2012). Towards a rational use of African rice (Oryza glaberrima Steud.) for breeding in sub-Saharan Africa. Genes Genomes Genom. 6, 1–7.
Sikirou, M., Shittu, A., Konaté, K., Maji, A., Ngaujah, A. S., Sanni, K., et al. (2018). Screening African rice (Oryza glaberrima) for tolerance to abiotic stresses: I. Fe toxicity. Field Crop Res. 220, 3–9. doi: 10.1016/j.fcr.2016.04.016
Singh, A., Carandang, J., Gonzaga, Z. J. C., Collard, B. C. Y., Ismail, A. M., and Septiningsih, E. M. (2017a). Identification of QTLs for yield and agronomic traits in rice under stagnant flooding conditions. Rice 10, 15–18. doi: 10.1186/s12284-017-0154-5
Singh, A., Septiningsih, E. M., Balyan, H. S., Singh, N. K., and Rai, V. (2017b). Genetics, physiological mechanisms and breeding of flood-tolerant rice (Oryza sativa L.). Plant Cell Physiol. 58, 185–197. doi: 10.1093/pcp/pcw206
Singh, A., Singh, Y., Mahato, A. K., Jayaswal, P. K., Singh, S., Singh, R., et al. (2020). Allelic sequence variation in the SUB1A, Sub1b and SUB1C genes among diverse rice cultivars and its association with submergence tolerance. Sci. Rep. 10:8621. doi: 10.1038/s41598-020-65588-8
Somado, E. A., Guei, R. G., and Keya, S. O. (2008). NERICA: The new rice for Africa – A compendium. WARDA, Cotonou, Benin.
Su, L., Yang, J., Li, D., Peng, Z., Xia, A., Yang, M., et al. (2021). Dynamic genome-wide association analysis and identification of candidate genes involved in anaerobic germination tolerance in rice. Rice 14, 1–22. doi: 10.1186/s12284-020-00444-x
Suvi, W. T., Shimelis, H., and Laing, M. (2020). Farmers’ perceptions, production constraints and variety preferences of rice in Tanzania. J. Crop Improv. 35, 51–68. doi: 10.1080/15427528.2020.1795771
Tanaka, A., Johnson, J.-M., Senthilkumar, K., Akakpo, C., Segda, Z., Yameogo, L. P., et al. (2017). On-farm rice yield and its association with biophysical factors in sub-Saharan Africa. Eur. J. Agron. 85, 1–11. doi: 10.1016/j.eja.2016.12.010
Thapa, R., Tabien, R. E., Thomson, M. J., and Septiningsih, E. M. (2022). Genetic factors underlying anaerobic germination in rice: genome-wide association study and transcriptomic analysis. Plant Genome 24:9262. doi: 10.1186/s12864-023-09262-z
Tsujimoto, Y., Rakotoson, T., Tanaka, A., and Saito, K. (2019). Challenges and opportunities for improving N use efficiency for rice production in sub-Saharan Africa. Plant Produc. Sci. 22, 413–427. doi: 10.1080/1343943x.2019.1617638
Ulzen, O. O., Buri, M. M., Sekyi-Annan, E., Devkota, K. P., Dossou-Yovo, E. R., Essel Ayamba, B., et al. (2022). Yield potentials of improved rice varieties for increased lowland rice production within the Mankran watershed in Ghana. Plant Prod. Sci. 26, 17–27. doi: 10.1080/1343943x.2022.2156893
UNDRR (2017). Nigeria releases AfricaRice “flood-tolerant” rice strains. Available at: https://www.preventionweb.net/news/nigeria-releases-africarice-flood-tolerant-rice-strains (Accessed February 24, 2023)
Valimba, P., and Mahe, G. (2020). Estimating flood magnitudes of ungauged urban Msimbazi River catchment in Dar Es Salaam, Tanzania. Tanzania J. Eng. and Technol. 39, 59–71. doi: 10.52339/tjet.v39i1.519
Van Oort, P. A. J. (2018). Mapping abiotic stresses for Rice in Africa: drought, cold, iron toxicity, salinity and sodicity. Field Crop Res. 219, 55–75. doi: 10.1016/j.fcr.2018.01.016
Vergara, G. V., Nugraha, Y., Esguerra, M. Q., Mackill, D. J., and Ismail, A. M. (2014). Variation in tolerance of rice to long-term stagnant flooding that submerges most of the shoot will aid in breeding tolerant cultivars. AoB PLANTS 6, 1–16. doi: 10.1093/aobpla/plu055
World Meteorological Organization (WMO) (2022). State of climate in Africa highlights water stress and hazards. https://public.wmo.int/en/media/press-release/state-of-climate-africa-highlights-water-stress-and-hazards (Accessed 23 February 2023)
Xu, K., and Mackill, D. J. (1996). A major locus for submergence tolerance mapped on rice chromosome 9. Mol. Breed. 2, 219–224. doi: 10.1007/bf00564199
Yamauchi, M., Aguilar, A. M., Vaughan, D. A., and Seshu, D. V. (1993). Rice (Oryza sativa L.) germplasm suitable for direct sowing under flooded soil surface. Euphytica 67, 177–184. doi: 10.1007/bf00040619
Yang, J., Wei, J., Xu, J., Xiong, Y., Deng, G., Liu, J., et al. (2022). Mapping QTLs for anaerobic tolerance at germination and bud stages using new high density genetic map of rice. Front. Plant Sci. 13, 1–15. doi: 10.3389/fpls.2022.985080
Zenna, N., Senthilkumar, K., and Sie, M. (2017). Rice production in Africa. Rice Production Worldwide, 117–135. doi: 10.1007/978-3-319-47516-5_5
Keywords: climate change, floods, smallholder farmers, rainfed conditions, flood-tolerant varieties
Citation: Mwakyusa L, Dixit S, Herzog M, Heredia MC, Madege RR and Kilasi NL (2023) Flood-tolerant rice for enhanced production and livelihood of smallholder farmers of Africa. Front. Sustain. Food Syst. 7:1244460. doi: 10.3389/fsufs.2023.1244460
Edited by:
Seth Etuah, Kwame Nkrumah University of Science and Technology, GhanaReviewed by:
José Manuel Gonçalves, Instituto Politécnico de Coimbra, PortugalKarl Kunert, University of Pretoria, South Africa
Benjamin Annor, Kwame Nkrumah University of Science and Technology, Ghana
Copyright © 2023 Mwakyusa, Dixit, Herzog, Heredia, Madege and Kilasi. This is an open-access article distributed under the terms of the Creative Commons Attribution License (CC BY). The use, distribution or reproduction in other forums is permitted, provided the original author(s) and the copyright owner(s) are credited and that the original publication in this journal is cited, in accordance with accepted academic practice. No use, distribution or reproduction is permitted which does not comply with these terms.
*Correspondence: Lupakisyo Mwakyusa, lupakisyomwakyusa01@gmail.com